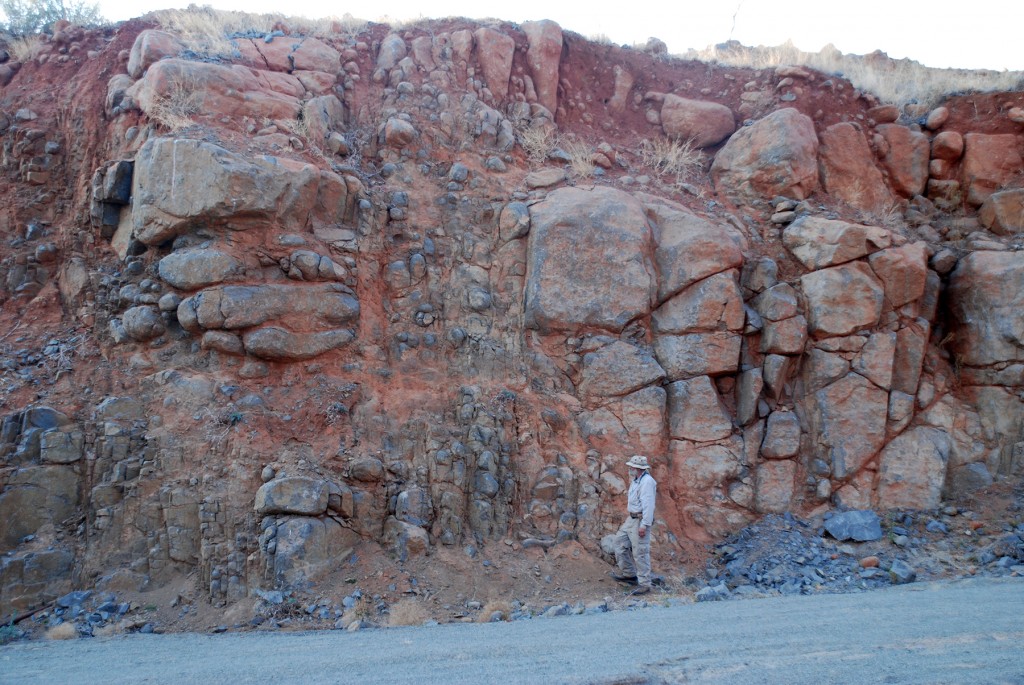
A quarry in southeastern Botswana exposes a weathering profile into a Mesoproterozoic sill of the Umkondo Large Igneous Province
This photo shows a weathering profile through a 1.1 billion-year-old mafic sill of the Umkondo Large Igneous Province that was exposed in a recently developed quarry in southeastern Botswana. My collaborator Richard Hanson is standing on fresh unweathered dolerite bedrock approximately 6 meters below the surface.
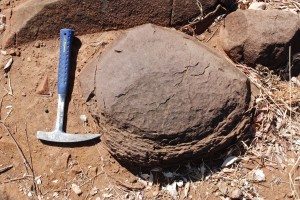
An example of spheroidal weathering where a corestone of fresh dolerite with only a thin oxidation layer is inside a zone of partially weathered rock layers called rindlets (Buss et al., 2008) giving the rock the appearance of an onion.
What can nicely be seen in the photo is that at the top of the profile near the surface are spheroidal boulders. These boulders have formed through spheroidal weathering (Chapman and Greenfield, 1949) wherein exfoliation caused by chemical weathering has lead to disaggregation of the rock that has progressed to make the sphere. The photo on the right shows an example of this weathering where a corestone of relatively fresh rock is surrounded by a zone of concentric, partially weathered rindlets (Buss et al., 2008). This process is indicative of expansion during weathering reactions that built up strain leading to the spheroidal fracturing. Further below the surface where Richard is standing in the main photo, there are corestones where the corners of pre-existing joint planes are being rounded off as a result of incipient spheroidal weathering. I was inspired to have a look at these photos yesterday by Prof. Susan Brantley of Penn State who was at the University of Minnesota giving a talk on observations of weathering and regolith development at Critical Zone Observatories in Puerto Rico and Pennsylvania. In the Luquillo Mountains site of Puerto Rico, quartz diorite is weathering spheroidally. They observe a relationship where the spheroidal boulders are smaller higher in the weathering profile but don’t have the excellent exposure given to us in this Botswana quarry. In the quarry, its clear that pre-existing fracture play a large role in determining the size of the weathering boulders. We do see, however, that the smallest most spherical boulders are just below the surface.
We were in Botswana to sample mafic sills like this one for paleomagnetic analysis. This work requires that we are able to sample fresh unweathered material where iron oxides that crystallized out of ancient magma have not been altered by recent surface oxidation. Spheroidal weathering works in our favor as the spheroidal fractures physically break off the chemically weathered rock leaving a core stone with a quite thin oxidation rind under which is unweathered rock with pristine iron oxide minerals. Yahtzee!
- Chapman, R. W., & Greenfield, M. A. (1949). Spheroidal weathering of igneous rocks. American Journal of Science, 247(6), 407-429. http://dx.doi.org/10.2475/ajs.247.6.407
- Buss, H. L., Sak, P. B., Webb, S. M., & Brantley, S. L. (2008). Weathering of the Rio Blanco quartz diorite, Luquillo Mountains, Puerto Rico: Coupling oxidation, dissolution, and fracturing. Geochimica et Cosmochimica Acta,72(18), 4488-4507. http://dx.doi.org/10.1016/j.gca.2008.06.020